Ask the pioneers: What’s next for materials science?
Expanded Interviews
At the beginning, we introduced various materials produced in Japan that have changed the world. How was Japan able to achieve such groundbreaking accomplishments? And what is necessary for Japan to continue churning out such results? In the face of mounting global challenges, the various roles to be filled by new materials will continue to increase. I asked the founders of Japan’s materials science to tell us based on their experience what Japan must do to retain its strength in materials science considering the limits on element resources.
Interviewer: Atsuko Tsuji
- 「Completely new materials will be discovered by humans, not AI」 Dr. Masato Sagawa, developer of the neodymium magnet
- 「Synergy among different fields will awaken Japan’s potential」 Dr. Akira Yoshino, developer of the lithium-ion battery
- 「It is essential to create an environment for tackling difficult challenges」 Dr. Hiroshi Amano, developer of the blue LED
- 「Creating a system that allows free rein to impertinent youth」 Dr. Hideo Hosono, developer of the IGZO semiconductor
- Final Thoughts Atsuko Tsuji
─How were neodymium magnets created?
Initially, I studied what makes a magnet, beginning with research on the samarium-cobalt magnet, which was commonplace at that time. In a magnet formed of the five elements samarium, cobalt, iron, copper, and zirconium, cobalt proved to be responsible for the magnetism. Normally, everyone would assume that iron is better, owing to its large magnetic moment. Since the role of rare-earth elements is to support magnetic properties, I realized that I could use something other than samarium. However, despite thinking iron was promising, everyone was convinced that magnets could not be made with rare earths and iron and so I gave up on the idea, but I never stopped wondering why it could not be possible. Around that time, I heard a lecture on rare-earth magnets that gave me the idea of incorporating boron in an iron alloy. I tested this theory at once with positive results. After measuring its magnetism, I realized that the new compound held promise as a magnet.
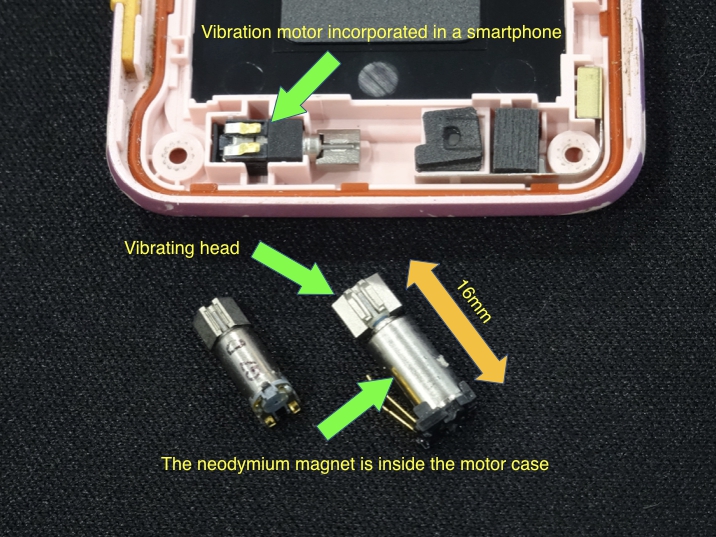
A vibrator for a smartphone.
The compact size is only possible with a high-performance neodymium magnet.
─How long did it take to reach the final result?
The experimentation part was faster than the calculation part. We created an alloy of samarium, iron, and boron, but the magnetic properties worsened despite the higher Curie temperature. So, we conducted various experiments using different rare elements and discovered that neodymium worked best. This took about a year of experimentation. It was another three years before we had any idea how to make an alloy of this combination into an actual magnet. Up to this point, my research had been conducted at Fujitsu, but the actual magnet was completed two months after I had accepted a new job at Sumitomo Special Metals (later merged with Hitachi Metals). Two weeks after we applied for a patent on the world’s strongest magnet, a similar application was filed in the U.S.
─That was very close timing, wasn’t it?
Even a slight delay, and we would have been too late. I was fortunate to have joined Sumitomo Special Metals, a materials manufacturer in my home region, after quitting my position at Fujitsu. We didn’t produce any materials at Fujitsu, and I had a poor relationship with my superiors. The president of the new company provided me with a large staff and told me I could have anything I needed. I created about 50 different composition tables and everybody tackled the work with enthusiasm. Two months later, we produced data unrivaled in the world. Having discovered the world’s strongest magnet, we studied the dependence of its magnetic properties on alloy composition and manufacturing conditions and extensively investigated basic data needed for its mass production. Three years after I had joined Sumitomo Special Metals, neodymium-iron-boron magnets were being mass produced. I think the company played a major role by making the snap decision to invest in me as a venture and facilitate my research. A system like the one I experienced, in which the company creates and operates a venture business, for example, is good for creating opportunities for carrying out research freely within a large corporation. Companies face what is widely known as the “innovator’s dilemma.” Improvements are made to the existing technology to increase profit, but no major changes are made. For this reason, I think the challenge is learning how to blend ventures with large corporations.
─Looking back, what were the factors of your success?
After receiving my doctorate in metals research at Tohoku University, I wanted to remain at the university. However, I didn’t write a very good dissertation and was not offered a position at the university, so reluctantly I went job-hunting. My first research task upon entering the company was to improve on the cobalt-based rare-earth magnet. Objectives were well-defined in company research, which I felt suited me well. I’m happy that I clung tenaciously to the idea that iron-based rare-earth magnets would surely have more benefit to humankind than cobalt-based ones because they are strong magnets that can be made inexpensively.
─Thanks to your early action, magnet research was expanded as a major area in the Element Strategy Initiative.
We started a new research area on iron-based magnets that was centered on two studies involving the neodymium magnet and a samarium-iron-nitrogen magnet discovered by Takahiko Iriyama at Daido Steel. We applied a deductive approach to advance from basic research to applications, expanding research into both industrial and academic areas, and I believe we produced some world-leading results. By making further developments in this direction, I hope that research in our area of iron-based rare-earth magnets will take the world by storm. Surprisingly, some of our results in studies for the Element Strategy Initiative have contradicted conventional theories on the role of boron in neodymium magnets, which has heightened the mystery.
─Are you saying that you still don’t know the role boron plays?
It is still unclear. My original idea was that the introduction of boron would expand the interatomic distance in metal and stabilize the ferromagnetic state of the crystal. Since elements that appear at the beginning of the periodic table, such as boron, carbon, and nitrogen, are small enough to occupy interstices of the crystal, I imagined that they could expand the crystal lattice. Whether this is true, I still don’t know. In any case, I was successful. Nevertheless, Professor Junjiro Kanamori, who was an authority on magnetics and physics research, explained that the role of boron is not to increase lattice spacing, but that boron electrons form a chemical bond with the iron electrons responsible for magnetism, thereby improving the magnetic properties of the iron. In other words, there is mutual influence among iron electrons in the 3d orbital and boron electrons in the p orbital. For a long time, I believed that explanation until recently Takashi Miyake (AIST) in research conducted for the Element Strategy Initiative removed boron virtually from a crystal formed of the compound neodymium, iron, and boron to create vacancies and, after calculating the resulting magnetic properties, realized that the magnetism was stronger without boron. If true, then we have to wonder what led to the previous explanation. When I discovered the neodymium-iron-boron magnet and created an actual crystal, I couldn’t have made it without boron, and yet I still don’t fully understand its role.
─It gives one a sense of profundity, does it not?
One cannot produce something entirely new through deductive thinking alone. I believe trial and error is also indispensable. The trial and error results I produced when creating the neodymium magnet would not have been possible in deductive research, building up from a fundamental, theoretical perspective. In the Element Strategy Initiative, Toshiyuki Shima of Tohoku Gakuin University recently discovered that magnetic performance improved after adding boron to a samarium-iron-cobalt magnet through trial and error, leading to the development of a new magnet. When I spoke to him about it, he said he was influenced by me. You won’t know unless you try. That is the way of materials. This research has the potential to grow significantly, and I hope we continue to place value on this type of trial and error approach.
─We are seeing a gradual shift in research due to the introduction of AI. Does it appear that AI will be of use?
I think AI is strongest under fixed rules, but there are no rules in research. AI only operates in the current sphere of development and cannot break out of that sphere. Accumulated research, also known as deductive research, is not sufficient to overcome barriers. You may have heard the term “backward induction,” where you work backward from a desired objective toward the principles. Such research requires intuition, which AI does not possess. AI is certainly powerful, but it is powerless to discover entirely new things, things we don’t already know. Only human researchers can do that. They may, for example, even discover a new magnet that does not use iron.
─What research are you currently engaged in?
An issue with neodymium-iron-magnets is their sensitivity to heat. The world’s strongest magnet that I first developed would lose magnetism at 50℃, and I was teased that it could only be used in toys. While working at Sumitomo Special Metals, I was able to make a practical version after learning that heat resistance could be improved through the addition of dysprosium, but dysprosium is problematic as an element strategy. So, we have continued research to eliminate the need for dysprosium and are on the verge of developing a new version that requires only a small amount. If this study proves successful, I think this phase of my research will be complete.
─What does Japan need in order to grow its materials research?
A research system and training capable of challenging research on the creation of a new “core” is essential above all else.
─Do you have some advice to offer young researchers?
Carry out your primary tasks but make good use of your free time. As a researcher, it is important to think, to think things all the way through. Do the research in your head. Try conducting actual experiments in your head. I was always thinking on the weekdays and would conduct experiments in the lab just one day on the weekend. Keep thinking about how you can meet the needs of society and change the world with your own efforts. What is needed to accomplish that? Continue to ask what can be done to achieve those goals, rather than insisting on what you can do. The needs may simply be the needs of basic research or the research required to develop basic research. I am concerned that research in Japan too often is focused on the researchers’ capabilities in what I like to call “how about this?” research and “let’s put that aside” research. I would like to see researchers, and not just young people, tackle these needs head on.
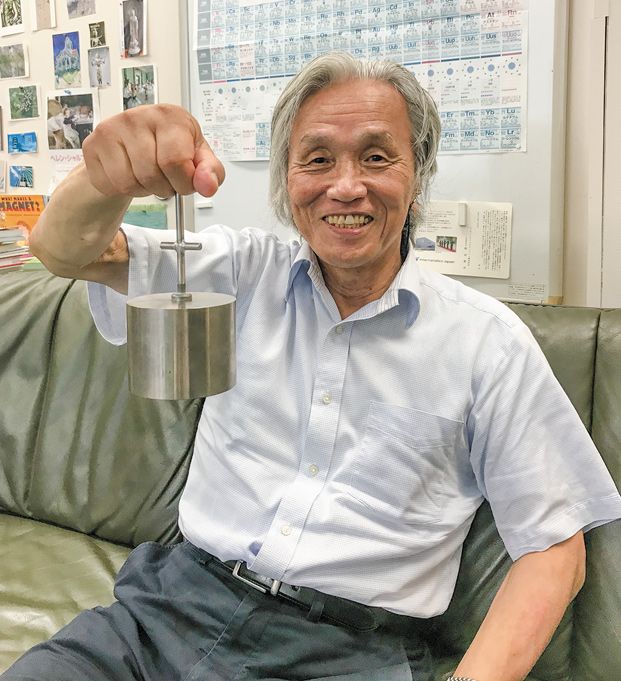
Masato Sagawa
An advisor for Daido Steel Co., Ltd.
who developed new materials for permanent magnets from a unique perspective, discovering a Ne-Fe-B (neodymium, iron, and boron)-based composition in 1982. Over the course of developing processing techniques, he perfected a composition for industrial use. Neodymium magnets are far superior to other types and have maintained their place as the strongest in the world.
─What were the main factors that led to the successful development of the lithium-ion battery?
Firstly, while compact batteries have been successfully developed in Japan, this success was supported by Japan’s strength in materials science. The main components of lithium-ion batteries are cathodes, anodes, electrolytes, and separators and can be seen as the culmination of new materials, which is Japan’s specialty.

Lithium-ion batteries for powering an electric-driven automobile.
Li-ion batteries have been adopted in a variety of devices owing to their light weight, high output, and rechargeability.
─It sounds like the original goal was not to develop batteries.
Historically speaking, a breakthrough occurred in the 1970s when Hideki Shirakawa developed polyacetylene. There was a mad rush worldwide to find applications for this new material. Initially I tried to ascertain the nature of the material and what products it could be used for with absolutely no thought of batteries. I investigated various uses for the material and whether it could function as a solar cell, conductive material, or semiconductor, for example. At that time, anode materials were a challenge in the development of new 2D batteries, and I learned that a new anode material was needed. That led me to connect the new polymer polyacetylene to its use as a battery material.
Knowing polyacetylene was discovered first in Japan was a great motivator. Moreover, it was rooted in the frontier electron theory of Dr. Kenichi Fukui. Polyacetylene has a metallic luster, and a single electron for each carbon atom. It has even been suggested as an alternative to copper wire. The movement toward an element strategy may also have been sparked by this research.
─How do you view the efforts of the Element Strategy Initiative?
Materials science has traditionally emphasized empirical science, but the Element Strategy introduced a computational science approach. If the data from computational science, data science, and empirical science do not agree, the experiments and computations are repeated. I think it is fascinating. I see strong similarities to the relationship between the polyacetylene developed by Dr. Shirakawa and Dr. Fukui’s frontier electron theory. I also think materials informatics has had a strong impact of late. Applying materials informatics along with experimentation should eventually lead to relevant materials. This type of approach is unique, and I believe it will become a strength of materials science.
─Research in materials science seems to be headed for a period of transition owing to AI and big data.
I think the important question is whether the big data that has been collected is of high quality. If the inputted big data is skewed, AI will provide incorrect answers. Particularly crucial is whether negative data has been inputted. Generally, data from positive results is preserved while negative data is not maintained and ultimately lost when the study is interrupted. However, it is necessary to accumulate examples of failures. Without such failure data, AI will never become intelligent. This is the essence of AI, and for that reason I have misgivings about AI in its current state. For example, data accumulated for the molecular design of anti-cancer drugs clearly specifies information like efficacy, side effects, etc. When such databases are shared, AI can easily be applied since mistakes in molecular design are known. On the other hand, data on lithium-ion batteries includes so many items, such as the temperature cycling tests were good but the high-temperature performance was bad, the high-temperature performance was good but the low-temperature performance was bad, etc., making it difficult to judge between good and bad. Another factor is that researchers want to produce good data and may neglect to input poor results. Having input that reveals such negatives would be immensely helpful.
Japan has overwhelmingly dominant strength in materials science and has accumulated much data from many past successful cases in particular. Undoubtedly, there is also much data on past failures, and I think it would be good if this were also inputted for AI, but it is difficult to collect this data once the research has been interrupted. There are no articles focused primarily on failures and those researchers that possess failure data from the past have already retired. We may need somebody with the determination to search these people out. Japan should have much more negative data than any other country.
─What must Japan do to further enhance its strength in materials science?
It’s essential to have processes for producing new technologies or processes for discovering new materials. Such processes will yield breakthroughs and, although the processes may not ultimately lead to commercialization, they are important because they will provide opportunities. To this end, we need researchers who can assess the properties of the materials themselves and the nature of their functions. The importance of ascertaining the nature of materials was important in my experience as well as it may ultimately lead to usable materials, even if the original materials do not prove useful.
We must use the synergy of experimentation and computation as two wheels for advancing research. Even if only one in a thousand researchers made an original discovery, the number would be quite significant when viewed on a global scale. However, if researchers in computations and experiments partnered up and each had original discoveries, the result would be one in one hundred through synergy. There are only one or two people in the world who can make discoveries on such a level. Through synergy such results become possible. However, synergy can only be achieved when the partners specialize in different fields. I think this manner of integrating different fields must be implemented over a wider scope.
This is also true for a novel coronavirus, for example. Both medicine and sociology are needed, and synergy is achieved with the science of infectious diseases and social systems.
─Sometimes the more refined the specialties of each field, the more difficult it can be to communicate.
It is precisely because of that difficulty that synergy works.
─Would the same be true between companies?
That’s an important point. The same effect is achieved between companies.
─Considering the big role that companies play in Japan’s materials research, as they did with your own work, getting companies involved is also a crucial issue, is it not?
There was a period in which Japan’s companies were vibrant. Even my research was made possible by a company. Carbon is the anode material of lithium-ion batteries, and I was fortunate that Asahi Kasei had a special carbon fiber, VDCF, on hand. The material had exceptional properties. Without this company and the use of their material, the battery may not have been developed.
It is also the reason that collaboration among industry, government, and academia is vital. Asking a university professor to identify something that will be useful ten years from now is unreasonable, but a company can clearly spell out what will be needed ten or twenty years from now. However, this is confidential information for a company because rival firms could try to duplicate the research should they get wind of it. Hence, companies in the industrial sector are the difficult aspect of industry-government-academia collaboration, and it is hard to know whether you can develop an honest relationship with them. For example, a company in Germany that has no competition in its areas of activity can collaborate openly with no problem. Japan has the disadvantage of having numerous companies that are difficult to coordinate and often compete needlessly among one other. And since government management is not desirable, they are difficult to control.
─You mentioned that occasionally something confidential to one’s own company is not necessarily so for another and something not particularly important to your company is sometimes important to another company.
That’s true in the majority of cases. I would like to see companies invigorate their common associations and interact through both bottom-up and top-down approaches under the condition that profits will be split if things go well. Creating synergy among fields and honest relationships between companies. If we can be a little more successful with this, I think we can waken Japan’s potential. There is a good chance that another company can find use for a technology that has not amounted to much at your company. Implementing this approach will be a future challenge for companies.
─What should be the future direction for the Element Strategy?
Currently, our main goal is to contribute to a sustainable society. The world is undergoing a transformation owing to information technology, but I think it all boils down to issues of the environment. If we focus solely on making the world more convenient, the people of the world cannot follow. Rather I have the sense that we don’t really need any more than what we have. To produce a global product, we must first consider environmental issues and then add perspectives of convenience and cost. I think the Element Strategy Initiative should aim toward technological innovation that will create a sustainable society.
─What steps can be taken to address the uneven distribution of resources, such as rare metals, that are commonly used as functional materials in batteries and the like?
Lithium and cobalt are problematic in the case of lithium-ion batteries. Handling the issue of rare-earth resources requires (1) production without use of rare metals, (2) recycling, and (3) sharing. As an example of sharing, battery cells operate only eight hours a day and are idle the remaining sixteen, but if the same cells have the capability of functioning for another purpose at night, costs can be shared. Preventing a device from idling in this way can help save resources. AI and IOT are needed to implement sharing. This could lead one to think that, if effective, sharing could be used even for somewhat rare elements. I believe this approach should be adopted as a national strategy.
─Do you have a message for young researchers?
This is a golden opportunity for you. Society has clear needs that humankind has not yet found solutions in relation to the global environment and infectious diseases, and technology has yet to catch up. There will be very few chances like this. If you can solve these problems, you will be esteemed around the world. The opportunity for researchers to flourish is here and now. The deadline of the SDGs is 2030. This is the ideal chance to become the only researcher in the world that contributed to the realization of a sustainable society.
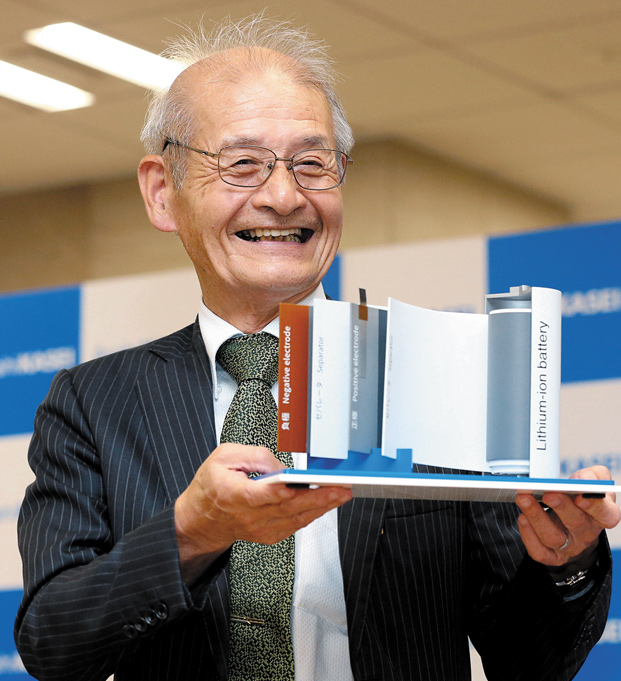
Akira Yoshino
Honorary Fellow, Asahi Kasei Corp. Professor, Meijo University.
In 2019, was awarded the Nobel Prize in Chemistry for developing the lithium-ion battery. Owing to their light weight, high-output, and ability to be recharged repeatedly, Li-ion batteries sparked the mobile age with its use in smartphones and other devices. More recently, they have been used in electric vehicles and have contributed to the realization of a society not reliant on fossil fuels.
─What were the main factors that led to the successful development of the blue LED?
The most important was the existence of Professor Isamu Akasaki, who led the way. Professor Akasaki initially worked on single-crystal growth of germanium under Tetsuya Arizumi, who had started up a lab for semiconductor engineering research at Nagoya University. Having graduated from the Faculty of Science at Kyoto University, Professor Akasaki was very fond of crystals. Later he transferred to Matsushita Electric Industrial, where he began working with gallium arsenide. It was during this period that Professor Akasaki became obsessed with light emission. Germanium does not emit light, but a wide bandgap semiconductor compound does, and he found this compelling. He chose to research gallium nitride (GaN) in the blue laser project sponsored by the former Ministry of International Trade and Industry. The quality of the crystals produced using an epitaxial growth method that required an ultra-high vacuum was poor, but after he shifted to vapor-phase epitaxy, the quality improved, as did its ability to emit light.

The development of blue LEDs, together with the existing red and green LEDs, completed the full set of primary colors, allowing for the expression of all colors and a wide variety of uses.
─He had emphasized that GaN was a unique material.
Professor Akasaki selected GaN for the solidity of its crystal. Compounds like zinc selenide (ZnSe) emit blue light, but its crystal is brittle and has a short lifetime. A wise researcher would be more likely to choose a II-VI compound that crystalizes at low temperatures and would probably not touch GaN, which requires temperatures higher than 1000℃. However, Professor Akasaki was not discouraged and dared to try despite the likelihood of failure. He had a strong enterprising spirit with the belief that the goal of research was to develop things people would use, and he never abandoned his fascination for the toughness of GaN. In the end, ZnSe could only illuminate for one hour, even though Sony and others later achieved light emissions of 100 hours, and research came to halt at that point.
─I understand you joined Professor Akasaki’s laboratory after he had transferred to Nagoya University.
After I was assigned to his laboratory, Professor Akasaki showed me a GaN crystal he had created during his period at Matsushita and I thought, “This is it!” I became obsessed with it, not knowing how difficult it would prove to be. I had false confidence that I could be the one to achieve success, but at that time I was probably more like the Monkey King in the Chinese novel Journey to the West throwing a fit in Professor Akasaki’s hand.
─And now you are working on the development of high-frequency, high-voltage devices using the same material?
The natural progression in the theory of semiconductors is that those with large bandgaps are suited for high frequencies, but not many people were working on power devices in those days. The high-frequency applications for GaN emerged around the same time that we learned of its long lifetime in blue lasers. Power devices and high-frequency devices also require the use of tough materials. Practical ultraviolet LEDs or lasers likely could not be realized outside the family of GaN, and GaN is also most promising for use in high-frequency devices since the device dimensions must be scaled down to increase frequency and power could not be produced without a high breakdown field. In my experience of manufacturing LEDs, GaN proved best from a productivity standpoint for devices handling high frequencies, but I think a variety of materials can all be used for different purposes.
Most GaN LEDs are being developed in the industrial sector. However, very little progress has been made around the world on applications for high-frequency and high-voltage devices, and I think universities have a lot more work to do than the industrial world. At the same time, social implementation will not progress with the attitude that companies must pull their weight after universities have done their part. In order to get companies to commit, universities must first take steps to demonstrate actual viability; in other words, show that a semiconductor can be operated any number of times or that a battery can be repeatedly charged. Companies will not initiate production without people willing to toil alongside them. I believe university research institutes must think things all the way through to innovation creation.
─In the Element Strategy Initiative, you have also been applying a coherent approach from theory to materials discovery.
Elucidating the mechanisms of functional expression and achieving that expression with a different element is a magnificent scientific and design technique. This is a splendid outcome that has enabled us not to become caught up in the scramble for rare elements and the hording by producing nations. On the other hand, substitution techniques have the potential for increasing costs by running up processing costs. In the future, I think it will be crucial to set comprehensive R&D objectives in coordination with the research system.
As for eventual applications, there are limitations to what a single material can do. For example, companies engaged in collaborative research developed a system using deep UV LEDs to inactivate coronavirus, but this required the development of not only LED chips but also various new polymeric and filtering materials that do not degrade under deep UV light. I would also like to use more materials in combination, rather than individual materials, in the Element Strategy to expand the number of applications. I would like to see more efforts toward encouraging new collaborations through information sharing.
─Gallium is also a rare element. What steps must be taken to resolve the problem of rare elements?
Certainly, China is currently responsible for most of the production of gallium, but when you look at the manufacturing process, gallium is produced by further refining bauxite, which is the primary ore of aluminum, and aluminum is in the same group as gallium. Thus, aluminum-producing countries that have large reserves of bauxite, such as Guinea and Australia, have the potential to become gallium-producing countries. However, these countries have traditionally discarded the gallium in bauxite residue, even though with refining technology it would be cost effective to create a business for producing gallium. There are likely other elements that have been needlessly discarded due to the nation not understanding their commercial value or not having the necessary refining technology, but this residue could be transformed into treasure. Japan should investigate global reserves and conduct business together with resource-rich countries while supporting them with refining technology. It will also be important to develop strategic reciprocal relationships with such countries, not only through cooperation from a technological and business standpoint, but also by building good relationships with them on an administrative level.
─Materials science is entering a period of transformation owing to advances in AI and data science, but what will be the key to future development?
I think databases and data servers are being constructed as we speak in a large number of fields, including fields of my specialty. To ensure their utilization, the databases and data servers must be more accurate and user-friendly for researchers. Various manufacturing processes including refining and reactions must be undergone in order to be able to use materials, but there is a limit on the number of times these experiments can be repeated. We could use people who have a talent for extracting optimal conditions in the fewest number of experiments and analyzing those conditions, and a software tool that can be utilized with a single click.
I believe finding a good method of use will be the impetus for steady growth. It is vital that we are the first to discover successful cases of things that were previously thought to be impossible. For that reason, it is crucial that we foster young competent researchers in every field.
─What will Japan need to achieve future growth in materials research?
Above all else, it will be important to create an environment for taking on difficult challenges, and particularly a system in which young researchers can feel secure in tackling such challenges. I’m convinced that there are no challenges that cannot be overcome by combining the best fields and personnel for the various projects, and I expect we will develop a strategy and organization that well enable us to compete with the rest of the world. I would very much like to create a system of project-based R&D focused on young researchers, whether currently in a doctoral program or within a few years after its completion, that enables them to move on to another challenge after finishing one project without having to worry about making ends meet.
─Do you have some advice for young researchers interested in this field?
In the past, Japan made do somehow by following in the footsteps of Europe and America, but you must be at the forefront of the world as leaders who will guide those in your field. Sustaining leadership is not possible without conviction in your own ideas and instincts. Mastering the basic skills is essential to developing self-belief.

Hiroshi Amano
Professor, Nagoya University. Director of the Center for Integrated Research of Future Electronics at the Institute of Materials and Systems for Sustainability.
Awarded the 2014 Nobel Prize for Physics together with Isamu Akasaki and Shuji Nakamura for the development of a blue LED from high-quality gallium nitride.
Currently working on developing next-generation power devices using gallium nitride.
─You have researched an astonishing variety of materials from IGZO amorphous oxide semiconductors to iron-based superconductors and synthetic ammonia catalysts. What is your secret to producing achievements in such diverse fields?
The key lies in electrons. The properties of a material are not determined by their elements alone. Structure is equally important because states of electrons determine the functions of the material, and elements and structure affect states of electrons. The key is connecting structure to function. I felt that there was no reason iron could not be used to make a superconductor and, while oxygen was a complete failure, I easily produced a superconductor with iron using a compound having a layered structure of arsenic and phosphorus. This surprised many people. It was unimaginable that glass could make a good semiconductor and, while other researchers were focused solely on crystals, I considered models showing good electron mobility even in amorphous materials and produced material designs of transparent amorphous oxide semiconductors (TAOS) of which one is IGZO. I used the well-known compound C12A7 made from lime and alumina, both components of cement, as an electride for a synthetic ammonia catalyst. While observing the electron states produced from this unique structure, I discovered new properties of electrons that led to the development of serviceable materials. So, while the materials I have studied are diverse, throughout I have focused on electron mobility.

A smartphone display.
As a core technology, IGZO accelerated the popularization of high-resolution displays.
─Your work seems closer to physics than chemistry.
I suppose my approach is to consider physics in terms of chemistry. The key is “tunneling,” whereby electrons pass smoothly through a barrier. Most oxides have barriers that electrons cannot move through but produce a tunneling effect and the structure suddenly becomes barrier-free. To me, uncovering a fascinating function by altering some commonplace material known for ages is what’s interesting about materials science. Unlike those who study condensed matter, I am not merely interested in the theory itself but am always considering how a material can be used in society. For a material to be useful, I look for chemical stability above all else, not simply good performance at instantaneous wind speed. Sometimes a candidate material is unusable if it absorbs moisture, for example, even if the properties of the material are good. If it can be used, it is a material.
─I suppose companies play a major role in what is used.
Various issues come to light when materials are manufactured on actual machinery at a production site. The laboratory level is not sufficient. Anybody can manufacture an IGZO transistor that elicits performance, but when we constructed and tested an actual display, it became clear that the properties of the transistors deteriorated when operated over a long period of time under a strong backlight. So, the company solved the problem by shielding the IGZO transistors through a clever method. The professionals in industry anticipate many things when creating something in earnest. We must develop materials that a company wants to use bad enough to work for them.
Another important element of material development is timing. Apply for a patent too soon, and the term of the patent may expire during the material’s period of utility. In the case of IGZO, it took about ten years to get from basic research on TAOS to the development of TFTs. I filed the patent application in 2004, which turned out to be ideal. In that period, demand had begun to rise for high-definition liquid crystal displays, but the performance of amorphous silicon transistors dominating the global market could no longer keep up. Thus, IGZO enabled the production if high-performance displays that amorphous silicon could not achieve. Hoping to work jointly with Japanese manufacturers to develop commercial displays, I took my idea to all of the major electrical manufacturers in Japan, as was recommended by JST and NEDO, but I was rejected. I was told the idea was premature or that I should develop the device with my own skills. In the meantime, my article was published in Nature, and I was immediately contacted by a South Korean manufacturer. At first, it was just part of their efforts aimed at information gathering, but they quickly became serious about initiating research when the TFT we trial-manufactured demonstrated the same performance detailed in the article. When the headquarters of Samsung Electronics, and not just the central research center of the Samsung Group (South Korea), gave a presentation on displays driven by IGZO-TFT at international conferences in 2008–2009, the entire business world was stirred to action. Sharp was next to adopt the technology. Such is the way of the industrial world. When Apple (U.S.) heard my presentation on IGZO at an international conference on displays, they commissioned and financed display companies to produce IGZO-based displays. Samsung, Sharp, and LG Electronics (South Korea) were some of the companies that produced commercial displays. Through the adoption of IGZO, the lifespan (evolution) of liquid crystal displays was extended from 2005 for another twenty years. And with their current use in OLED displays, I guess in a sense IGZO-TFTs have dominated the global display market.
TFTs manufactured with IGZO have an operating temperature as high as 200℃ with an extremely small leakage current and have begun to branch off in an entirely different direction from amorphous silicon, such as in applications for memory. The semiconductors are being eagerly researched by Toshiba, Samsung, and others with an accelerated effort, since materials like IGZO with previously demonstrated practical use have earned the trust of companies. Companies have no confidence in materials that have only appeared in journals such as Nature and Science, but they develop trust quickly once the material has been commercialized.
─Is the aim of the Element Strategy Initiative to develop materials that companies will take seriously?
Yes. It is important to develop new scientific concepts. In the Element Strategy Initiative, foundations were consistently established on new science, making our jobs easier by enabling us to conduct research on our own ideas, without having to demonstrate substitutes for rare elements, and a lot of interesting ideas have emerged. We are also fortunate to have a ten-year period for research, although ultimately we approach our research with the determination to create an achievement that will benefit society. This pursuit is expected to realize materials that can be produced worldwide without reliance on rare elements, which is the objective of the Element Strategy Initiative. In the end, that can be accomplished with new ideas and new scientific concepts.
Companies spend great effort on things that are profitable. Thus, while these efforts are not made public, the companies are making significant advances on many things through internal research. University researchers cannot easily compete in these areas. I would like to see us build more relations with such corporate researchers. By getting to know more company researchers, students and postdoctoral researchers could learn more about these companies, which I think is crucial to invigorate researchers at universities as well as companies.
─Universities and companies both have their own role to play, don’t they?
Universities have the advantages of novel subject matter and the youthful energy of students. The power of graduate students is particularly amazing. They will conduct experiments all night on New Year’s Day, even when you tell them not to. I admire that. In my experience, ideas often come to me in the middle of the night, and I can’t wait for daybreak to try them out. More often than not the results are not good, but at least the research is fun, and that anxious feeling of waiting for the sun to come up is more important than whether or not your idea will become the subject of an article. These days when I go to the university early to conduct an experiment, a student will say something like, “I’m working here, Professor. Get out of my way.”
─Research seems to be changing due to developments in AI and data science. Does it appear that they will be of use?
People like us who are aiming to produce new buds do not have very high expectations for today’s data science. Data cannot yet be used to generate the core of research or to create a new seed. It is possible that AI could be used to formulate hypotheses. My approach to research begins from a hypothesis. It doesn’t matter whether that hypothesis is always correct, but it provides a guide for taking steps in a certain direction. With a guide, one can set an axis and take off, and if the direction proves wrong one can make adjustments. However, I think it could be useful to check hypotheses produced through AI with one’s own.
─What is important for Japan to achieve future growth in materials research?
You can’t conduct materials research among only researchers in the same field. It is necessary to cross boundaries and break molds, and we need a system and funding to do that. Graduate schools also must remove restrictive divisions and broaden academic classifications for students. They must create a system not based on social order. In other words, single out the researchers who are motivated and talented and let them make their own decisions and work as they please. I would like to create an environment in which young people can thrive without being coddled. Once during a JST project, I asked a researcher the significance of their study, to which the youth replied, “It’s not as simple a problem as the professor thinks.” That is the type of impertinence I am looking for. I would rather have them tell me, “you may not understand this, but…” rather than have them present something that previous generations can easily follow. There is no need for them to think they have to act in a certain way. That’s how I would like element strategy research to be carried out. Naturally, it’s also important for the senior researchers to rise to the challenge and compete with their younger counterparts.
I have taken it upon myself to create such a system at the Materials Research Center for Elements Strategy at the Tokyo Institute of Technology. By having overseas professors primarily from Asia take up residence and by adding a mix of overseas students, I am trying to promote internationalization in the true sense of the word. Professor Hidetoshi Fukuyama has also facilitated the use of large-scale research facilities, such as the accelerators at the High Energy Accelerator Research Organization. Now young researchers are going to and from Tsukuba and using these facilities routinely, which has greatly encouraged the exchange of ideas among these young people. Ten years ago, I would have never thought that these facilities could be used so commonly. Having the opportunity to share viewpoints with one another I think is a great asset for young people. I also ensure that we assemble once each month, rather than every six months, to discuss outcomes of the project. Otherwise, they cannot share information based on fresh data and ideas. Naturally, confidentiality is strictly maintained. It would also be problematic to wait as much as half a year to learn of unsuccessful outcomes. For that reason, it is important to maintain close physical distance.
─Do you have a message for young researchers interested in this field?
I am certain that you can create new materials. If you dig while thinking carefully and observing well, you will find water. And if you don’t find water, you’re not digging deep enough. Always keep in your heart the desire to be useful to society.

Hideo Hosono
Institute Professor and Honorary Professor at the Tokyo Institute of Technology. Director of the Materials Research Center for Element Strategy.
Produced such outstanding research achievements as the creation of iGZO semiconductors used in LCD and OLED TVs, the development of a method to synthesize ammonia at low temperature and pressure using electrides expected to have a ripple effect throughout the energy industry, and the discovery of an iron-based superconductor that defies conventional wisdom.
Interviewer’s PostscriptFinal Thoughts
These interviews made me realize once again the important role that companies play. Drs. Sagawa and Yoshino talked about the indispensable assistance they received from companies, and Dr. Akasaki’s corporate experience also proved to be key. Thus, the importance of industry-academia collaboration in the true sense of company involvement is an opinion shared by all. They also talked about the need for university researchers to take a step forward and the company-side to have an eye toward technology. I look forward to the Element Strategy Project creating more opportunities for collaboration in order to produce more world-changing achievements.
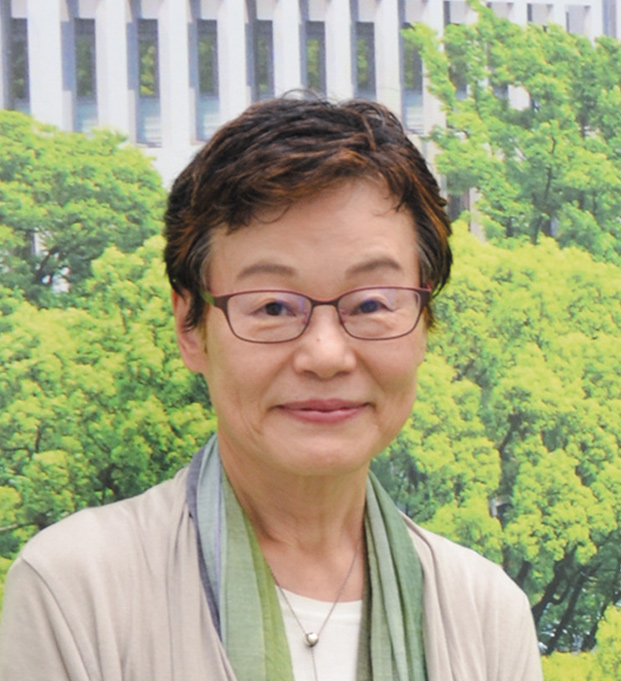
Atsuko Tsuji
Professor, University Research Administrator Organization, Chubu University.
Was engaged in science-based reporting at Asahi Shimbun and, from 2004 through 2013, was an editorial writer in charge of editorial articles on science and technology and medicine. Became a designated professor for Nagoya University International Organization in October 2016. For three and a half years, published Nagoya University Watch on the university’s website showing the university from a journalist’s perspective. Nagoya University also published a paperback edition with the same themes. Was appointed to her present position in June 2020.